January 29, 2014 report
Photochemical imprinting of neuronal activity: A flash memory for spikes
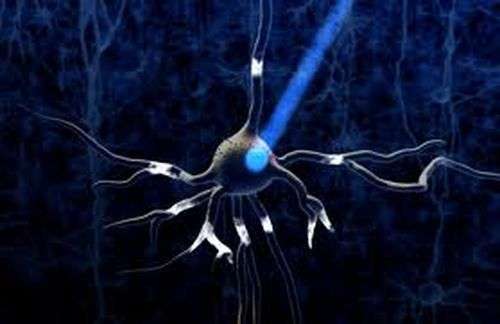
(Medical Xpress)—Animals experiments primarily serve two main functions. They give us insight into how biological systems might work, and they also act as test beds for treatments and devices we want to use on ourselves. In neuroscience, particularly with regard to large scale recordings, what we are ultimately interested in is correlating activity maps with thoughts. Cutting to the chase, the thoughts we really care about are not those of animals, or even so much those of other people, but actually the thoughts we generate ourselves. If we are to help a paraplegic do much more than kickoff the World Cup using an electrode pincushion-controlled exoskeleton, we can't simply infer some else's thoughts to generate algorithms for a machine, rather, we must first be able to infer our own.
When we have that capability, any residual posturing with animal experiments should rapidly draw to a close. In the meantime, a few new advancements that bring us nearer to that dream, are particularly noteworthy. Earlier we discussed Ed Boyden's work on light field microscopes, and hinted that more sophisticated sensors that track rapid changes in voltage, as opposed to slower calcium dynamics, are needed to fully utilize the power of the technique. A group of Harvard chemists just published a paper the Journal of the American Chemical Society that describes their progress toward that end. I asked corresponding author Adam Cohen a few questions about their research, and he wisely asked that I don't hype the work as the answer to brain activity mapping.
Hopefully the intro here did not mislead. I will point out that the title used for Adam's paper did say something about a "Flash Memory" for imprinting activity inside neurons. The flash here is obviously not a flash drive shrunk down and put into neurons. It refers to a voltage sensing protein that can be toggled between various stable states, and equilibria, using different wavelengths of light. A "write" state can be turned on, held, and then turned off, by a flash of light to accumulate voltage information. Later, even up to 2 minutes later according to Adam, another beam can be patched in using a tunable acousto optic filter to put the sensor into a "read" state that fluoresces.
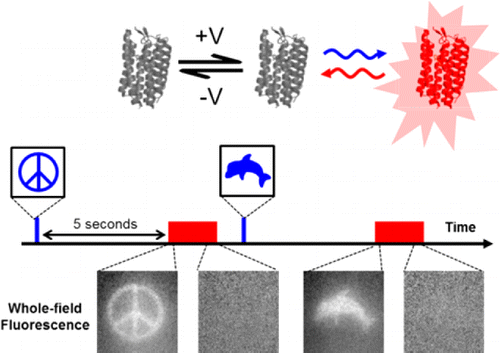
Pretty exciting stuff, but for the moment at least, the sensors are not quite as fast or sensitive as we might hope. The technique though, may provide an important bridge from the cruder sensors we use now, to the indefinite point in the future where exotic molecular tickertapes or activity barcode technologies actually reach the stage of rigorous, techno-economic discussions on bioRxiv.
Recording signals from the brain is really a two part problem. The first part is sensing and capturing the activity, and the second is getting that signal out of the skull. Tickertapes are a great way to achieve the first, but offer little as to the second. Flash memory devices, on the other hand, can directly address one looming hard reality of the business: while the recording problem may be local, small, and fast, reading the signal out from a massively parallelized, instrumented brain, may require significantly more time. As we have seen in the light field microscope, time is of the essence, especially if you are trying to run massive deconvolution threads in real time.
What the flash memory concept provides is a way to take a snapshot of activty, and then freeze it while you read it out. The Harvard group developed at least two distinct sensors, one that acts as a "sample-and-hold" device, and one that uses an "integrator" mode. In an ideal sample-and-hold the indicator reports the voltage at the exact moment the light is turned off. Adam says that they are pretty close to that, but that there is some blurring due to finite kinetics of the reporter. The integrator mode generates a stepped signal, perhaps more analog in actuality, that reflects accumulated spike activity. We should probably note here that single spike resolution would be difficult to extract from the integrator at this point.
To make the technique work well in neurons a bit more work needs to be done as far as targeting the proteins to proper spot in the membrane. Voltage sensors report the potential difference across the membrane and won't generate a signal if just floating around a conductive cytoplasm. For now, the group is using an HEK cell line that they engineered to spontaneously generate spikes just like neurons. By expressing sodium and potassium channels in these cells they can get spikes with fairly good rise times (~4ms) but then a more modest ~300ms duration and ~200ms refractory period before firing again. Presumably the cells have some endogenous ion puming capability, but probably not the full capabilities of neurons. The sensor proteins themselves where found by expressing a library of archaerhodopsin 3 mutants in the HEK cells and then screening for sufficient voltage-sensitivity.
Expressing tools in specific sets of cells that can activated, deactivated, or inactivated entirely with light may be powerful, but everything we need for a successful technology. Boyden's group has been developing some interesting new devices to help bring our optical neuroheritage to fruition. Critical to this goal are microstructured light guides which can be used for precision light delivery. While it may be possible to generate light deep inside brains from an electrical supply, it would be difficult to generate carefully metered pulses with tightly controlled properties without a fair degree of bulk.
By using advanced microfabrication materials and processes, (SU-8 photoresist, silicon oxynitride, and silicon dioxide, etc.) Boyden's group can create specific 3D geometries of varying stiffness so that they can adapt to natural deformations within the tissue. They are also working of their own light-controllable sensors, in particular, one they call lumitoxin. Illumination would cause this chemical photoswitch to unfold, deconcentrate from the cell surface, and release potassium channels from inhibition. Getting these new kinds probes to work in harmony with neurons, without stressing or poisoning them will be critical for translating this technology one day into humans. "Lumitoxin" may not be the perfect brand for a successful marketing strategy, but with proper investments and focus many may see the fruits of this work in their lifetime.
More information: Flash Memory: Photochemical Imprinting of Neuronal Action Potentials onto a Microbial Rhodopsin, J. Am. Chem. Soc., Article ASAP. DOI: 10.1021/ja411338t
Abstract
We developed a technique, "flash memory", to record a photochemical imprint of the activity state—firing or not firing—of a neuron at a user-selected moment in time. The key element is an engineered microbial rhodopsin protein with three states. Two nonfluorescent states, D1 and D2, exist in a voltage-dependent equilibrium. A stable fluorescent state, F, is reached by a photochemical conversion from D2. When exposed to light of a wavelength λwrite, population transfers from D2 to F, at a rate determined by the D1 D2 equilibrium. The population of F maintains a record of membrane voltage which persists in the dark. Illumination at a later time at a wavelength λread excites fluorescence of F, probing this record. An optional third flash at a wavelength λreset converts F back to D2, for a subsequent write–read cycle. The flash memory method offers the promise to decouple the recording of neural activity from its readout. In principle, the technique may enable one to generate snapshots of neural activity in a large volume of neural tissue, e.g., a complete mouse brain, by circumventing the challenge of imaging a large volume with simultaneous high spatial and high temporal resolution. The proof-of-principle flash memory sensors presented here will need improvements in sensitivity, speed, brightness, and membrane trafficking before this goal can be realized.
© 2014 Medical Xpress