April 21, 2014 feature
What you see is where you go: Fruit fly visual interneurons may compute temporal integration of visual motion
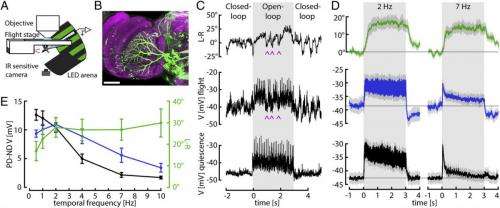
(Medical Xpress)—The fruit fly Drosophila melanogaster is a model organism (a non-human species that is extensively studied to understand particular biological phenomena) studied in a wide range of laboratory experiments for several reasons, including being easy to grow, presenting various visible congenital traits, and having an extremely large salivary gland chromosome. In particular, studies of how neural circuits process sensory information make use of Drosophila's visually-driven behaviors as a model system. Recently, scientists at the University of Washington have demonstrated that one of the computations performed by this system is temporal integration of visual motion. Moreover, by measuring the activity of identified visual interneurons during tethered flight, they describe the way this computation might be performed.
Dr. Bettina Schnell discussed the paper that she and her co-authors published in Proceedings of the National Academy of Sciences, first addressing the main challenges the scientists faced in showing that one of the computations performed by the visually-driven behavioral system of the fruit fly, or Drosophila, is temporal integration of visual motion – that is, the optomotor responses of flying Drosophila follow a time course consistent with temporal integration of horizontal motion input. (As stated in the paper, in the optomotor response, flies compensate for deviations from a straight flight path by turning in the direction of large-field visual motion.) "We only started to think of temporal integration within the optomotor system after we saw that the responses of the horizontal system visual interneurons, or HS cells, followed such a different time course from the behavior," Schnell tells Medical Xpress. "This was surprising, as HS cells have always been thought to control this behavior."
The historical challenge has been that measuring HS cell responses during flight has only become possible very recently, she explains. "In contrast, optomotor turning behavior in tethered flying flies has been studied for many decades now, which makes you think that everything is already known about it. However," she adds, "looking at it from an engineering perspective, temporal integration in this system makes a lot of sense, as it plays a key role in engineered feedback control systems."
Schnell turns to the task of providing evidence of how this computation might be performed by measuring the activity of identified visual interneurons during tethered flight that are thought to control the described behavior. "We saw that although HS cell responses look different during flight compared to rest, their membrane potential does not reflect a temporal integration of visual motion – so we knew that integration must happen either at the level of downstream neurons, or at the terminals of HS cells." Since calcium plays an important role in many neuronal computations, the researchers wanted to investigate whether calcium concentration in HS cell terminals follows a time course similar to the cells' behavior. "We were lucky in the sense that we found signals that resemble the behavior at that level," Schnell notes, "since we're only now learning how to record from downstream neurons."
The scientists used a genetically-encoded indicator to find calcium accumulates in interneuron terminals along a time course consistent with the behavior – experiments that were only possible, Schnell stresses, due to recent improvements in genetically encoded calcium indicators with much faster time constants than previous versions, thereby allowing them to draw conclusions about the actual time course of calcium accumulation. "One challenge of these experiments is that the fluorescence during rest and when the cells are not stimulated is relatively low," Schnell explains. The result was that they could only see cell terminal arborizations (multiple branching at the end of a neuron's axon) clearly once the cells were activated. "Another challenge," she adds, "is brain motion, which can make the terminals move out of focus. This was a particularly critical obstacle because the flies we used in these experiments were alive and flying."
The researchers propose that calcium accumulation provides a mechanism for temporal integration of sensory feedback consistent with the elimination of steady-state error through what is termed proportional-integral control, or simply PI control. "We were somewhat worried that the fluorescence signals we measured might not reflect true calcium accumulation," Schnell tells Medical Xpress, "but instead might be an artifact of the relatively slow time constants of the calcium indicator. We therefore performed several controls to show that this is not the case, modeling responses based on the reported time constants and assuming that there was no temporal integration." However, she acknowledges, those predicted responses could not explain the signals they actually measured. Moreover, they also imaged the cells presynaptic to HS cells, which do not temporally integrate the stimulus, and those responses also appeared different from the responses we measured in HS cells. "We're now reasonably confident that our results provide evidence for calcium accumulation in the terminals of HS cells," she concludes.
A difficult part of their research was performing whole-cell patch-clamp recordings from the set of HS cells thought to control the optomotor reflex during tethered flight. (In whole-cell patch-clamp recordings, currents through multiple channels are recorded simultaneously over the entire cell membrane.) "Performing whole-cell patch-clamp recordings in a living fly is not easy," says Schnell, "because you have to locally remove the neurolemma" – a thin membrane consisting of a matrix of proteins and glial cells that forms a sheath around nerve fibers and surrounds the brain. "Since you otherwise, wouldn't be able to form a tight connection between the electrode and the membrane of the cell you want to record, a so-called giga seal that is essential for a good recording." She adds that while HS cells have the advantages of having comparatively large cell bodies and of being relatively superficial, the biggest challenge is to avoid doing too much damage to the fly to make certain that so that they can still fly and show behavioral responses to the visual stimuli.
In addressing these challenges, Schnell points out, their work built on several recently-developed techniques – primarily the preparation that allows performing physiological recordings from tethered flying flies. "This preparation was developed by a former postdoctoral researcher in the lab, Gaby Maimon1," Schnell says. "We were, however, one of the first to use this preparation to directly compare behavioral and neuronal responses in the same flies."
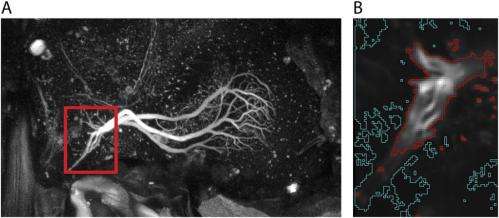
One of the paper's key findings is that presynaptic calcium accumulation in interneurons mimics a so-called leaky temporal integration of the visual motion signal. (Leaky in this context refers to a mathematical computation of temporal integration in which the signal decays over time, whereas a true integration would mean that all of the previous stimulus history would be relevant. That, in turn, would mean that if there was no additional stimulus, the signal would stay at the same level forever – which is not what the scientists found.) Biophysically, notes Schnell, this means that if calcium accumulation in presynaptic terminals is indeed the major mechanism for temporal integration, then calcium will still be slowly cleared from the terminals.) "Calcium has many different neuronal functions," Schnell explains. "At the synaptic terminal it plays a role in transducing an electrical signal, the membrane potential, into a chemical signal – that is, the release of neurotransmitters. An increase in membrane potential triggers the opening of calcium channels and leads to influx of calcium ions into the cell. This increase in calcium in turn triggers the release of synaptic vesicles and thus neurotransmitters, which activate postsynaptic neurons."
Usually, calcium is cleared from the terminals pretty quickly – either because it binds to buffers within the cell, or by being extruded via pumps. "Our findings suggest that in HS cell terminals, mechanisms of calcium clearance act slowly, which allows calcium to accumulate," Schnell adds. "We speculate that this calcium accumulation causes prolonged transmitter release and thus activation of downstream neurons. If that's true, the responses of downstream neurons should look more like the behavioral responses than the HS cell responses – but this remains to be seen."
The scientists propose that the genetic tools available in Drosophila will enable studying the precise mechanism of temporal integration in this model system, which could provide a number of insights into general mechanisms of neuronal information processing. According to Schnell, these tools will allow the scientists:
- to label and record from downstream neurons and confirm that their responses are a temporally integrated version of the stimulus
- attempt to interfere with calcium signaling in HS cell terminals by expressing calcium buffers or calcium channels and see how this affects the behavior, in order to prove that calcium accumulation is the main mechanism for temporal integration
- investigate how the synapses in HS cells differ from those of other neurons that do not perform temporal integration by checking which types of calcium channels or buffers are expressed in these cells
"While all these things are theoretically possible, they are all very challenging and might have their caveats," Schnell points out. "For example if you interfere with calcium signaling in a cell you might disrupt other processes than integration. However, temporal integration is likely a computation that has to be performed in a variety of different systems and organisms – and we speculate that mechanisms uncovered in Drosophila might also be implemented in the nervous systems of other organisms."
Moreover, the paper notes that extensive changes in wing stroke amplitude could be required in free flight to compensate for the imbalance created if one of the wings would be even slightly damaged, a function in which PI feedback would likely play an essential role. "Another recent paper from our lab shows that the actual changes in left and right wing stroke amplitude are relatively small when flies are performing maneuvers in free flight," Schnell explains. "We've wondered why flies have the capacity to perform such large changes during tethered flight – and one hypothesis is that flies have the capacity to generate large asymmetries in wing motion in order to compensate for wing damage." For example, she illustrates, damage of one wing would create a large imbalance in force, and if a fly wasn't able to compensate for that imbalance it would spin out of control or continuously fly in circles. "By strongly increasing the wing stroke amplitude of one wing flies would be able to compensate for this imbalance and fly straight. We further think that temporal integration is necessary to fully compensate for any imbalance."
Describing the planned next steps in their research, Schnell says that the scientists are currently trying to record from cells that might be postsynaptic to HS cells using electrophysiology and calcium imaging to determine if their responses show stronger correspondence with the optomotor response. "These cells are descending interneurons that receive input in the brain and project to the thoracic ganglion," she explains, "where they make synapses with motor neurons that directly control wing motion. If our hypothesis is correct, some of these cells should show responses that look like the temporal integral of the motion stimulus similar to the behavior."
In addition, Schnell continues, changes in wing stroke amplitude are only a part of the flies' behavioral repertoire for performing steering maneuvers. "We'd like to be able to measure other aspects of steering behavior, such as the deviation or angle of attack of the wing, or abdominal movements in tethered flight – and we're currently trying to develop tools to do that."
Schnell points out that there are other areas of research that might benefit from their study. "Elucidating the cellular mechanisms for temporal integration in Drosophila might provide insights into how brains in general perform these types of computations. It might therefore eventually help our understanding of how the brains of more complex organisms like mice, chimpanzees and even humans work. In addition, since our research takes us one step further in understanding how the optomotor response allows flies to control their course during flight, in the future this work might help engineers create autonomous flying vehicles which could be beneficial in a variety of applications."
More information: Cellular mechanisms for integral feedback in visually guided behavior, Proceedings of the National Academy of Sciences, April 15, 2014 vol. 111 no. 155700-5705, doi:0.1073/pnas.1400698111
Related:
1Active flight increases the gain of visual motion processing in Drosophila, Nature Neuroscience 13, 393–399 (2010), doi:10.1038/nn.2492
© 2014 Medical Xpress