Sizing up bone growth: A surprising cellular mechanism drives skeletal proportions
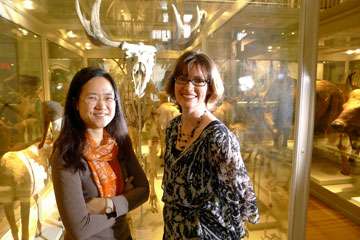
(Medical Xpress)—Stroll through the Harvard Museum of Natural History and gaze up at the whale skeleton looming overhead. Look down at the furry foxes curled up inside their glass display cases. Don't forget the bat with shadowy wings spread like a delicate shawl. They are all mammals, but their body proportions are so distinct one can tell them apart just by glancing at their calcified skeletons.
Each animal's skeleton is a collection of differences, too, with bones of varying sizes from their long limbs to spindly toes. Kimberly Cooper, an HMS research fellow in genetics interested in the diversity of skeletal morphology, studies how these skeletal components grow. In particular, she focuses on the three-toed jerboa, the "T. rex of the rodent world" in anatomy if not temperament. It stands upright on dramatically elongated hind legs that dwarf its stubby forelimbs. But how did it get that way?
In a paper published online March 13 in Nature, a team led by Cooper and Seungeun Oh, an HMS research fellow in systems biology, begins to answer that question. Applying specialized microscopy techniques to the problem, they discovered a surprising cellular mechanism that drives bone growth in jerboas and their quadripedal cousins, mice, with the potential to also illuminate human biology.
The cells that contribute to lengthening of bones, called chondrocytes, are actually cartilage cells found in the bone's growth plate. Whether they are femurs in the thigh or metatarsals in the foot, all limb bones start as rods of cartilage that create a scaffold onto which calcium is laid down. Cooper's central question about differences in bone growth led her to investigate growth that comes not just from cells proliferating—dividing again and again—but from cells enlarging.
In order to explore this, Cooper needed to measure single cells. Through Cliff Tabin, HMS George Jacob and Jacqueline Hazel Leder Professor of Genetics, head of the Department of Genetics and a senior author of the paper, she connected with Oh, who had recently brought her expertise in biomedical optics to the lab of Marc Kirschner, HMS John Franklin Enders University Professor of Systems Biology, head of the Department of Systems Biology and a senior author of the paper. Oh adapted a traditional technique to a new purpose: measuring mass in cells by using a quantitative phase microscope, or QPM.
The principle of measuring a live cell's dry mass using light microscopy was introduced in the 1950s. It is based on the linear relationship between an optical property—the refractive index—and the concentration of macromolecules. A higher concentration of macromolecules increases the refractive index of the cell, which in turn makes light travel through the cell more slowly. This results in retardation of light's wave front in proportion to the quantity of macromolecules in the cell. QPM uses interferometry to quantify the wave-front change caused by the cells from which the total macromolecule content in single cells is calculated.
The researchers measured the growth of the single-chondrocyte population by two different quantities: by volume and by dry mass (macromolecule content). Surprisingly, the rate of mass increase differed from the rate of volume enlargement. Together they showed three distinct stages of cell growth.
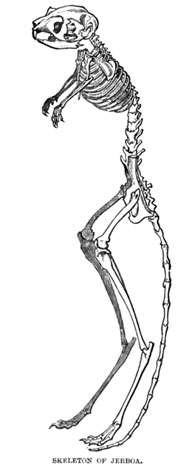
In the first phase, the initial high density of the dry mass is maintained. That is followed by a swelling phase, when individual chondrocytes enlarge with extra fluid. In the third phase, size continues to increase while low density remains constant.
Such swelling is well known in plants, which take up water as part of their growth, but it is surprising in mammals. Most animal cells have finely tuned controls that keep fluid levels constant. The volume increase Cooper and Oh documented in rodents is rapid, lasting only about 12 hours.
"That's extraordinarily fast," Cooper said. "We think that's where the swelling mechanism might be beneficial. A cell has to put energy and time into making proteins, lipids and carbohydrates. If a cell can shift the balance of fluid relative to these other things it is making, then the fluid can do the work of getting bigger much more easily. It's more bang for your buck."
The speed of chondrocyte swelling reminded Cooper of a 1989 review of bone growth. Some Iowans say they can hear the crackling of cornstalks growing as they walk through cornfields at night, wrote University of Iowa researchers Joseph A. Buckwalter and Richard D. Sjolund. They compared a child's rapid bone growth to this remarkable process in plants, making Cooper wonder about a child's sensation of "growing pains."
In the third step of the chondrocyte enlargement process, the fluid uptake again becomes proportionate to the rate of dry-mass production, such as proteins, lipids and carbohydrates, and cell swelling ceases. The duration of this final phase of proportional cell enlargement after swelling varies the most between bones—tibia in the leg and radius in the arm—and between mice and jerboas.
While the three stages of cell enlargement provide a framework for better understanding bone growth, it raises more questions, too, Oh said.
"It surprises me that chondrocytes dilute the cytoplasm to attain their large size," she said. "We want to find out what mechanism underlies the autonomous swelling of these cells."
The third stage also contained a surprise: This final phase appears to be regulated through a mechanism that depends on insulin-like growth factor (IGF-1), a major player in mammalian growth that is found throughout the body. Mice that lacked IGF-1 in their limbs not only had smaller limbs, but the cells in their tibiae and metatarsals were the same size. Usually tibia cells are bigger to begin with than metatarsal cells, in part contributing to the differences in growth rate. That suggests IGF-1 also plays an important role locally to regulate proportionality differences.
"It could be that IGF-1 is a really important regulator in what controls the difference in size of cells in different growth plates," Cooper said. "That's a future direction for research."
The three distinct phases of cellular enlargement is a novel observation, said Cornelia Farnum, a Cornell University College of Veterinary Medicine emerita professor of anatomy who has studied differential bone elongation. Her work is cited in the Nature paper.
"For over 50 years people have documented chondrocytes' increasing volume during hypertrophy, and how the final volume correlates positively with rate of elongation. But the how of that has not been cracked," she said. "The idea that there are regulatory control points within enlargement during hypertrophy is something that is new and begs to be studied. In the end you hope to find something that might be significant for understanding dwarfism or gigantism in humans."
Cooper and Oh hope their work will explain mysteries beyond the museum's hall of mammals, with implications for bone growth and treatment of limb length asymmetries in people.
More information: dx.doi.org/10.1038/nature11940