September 5, 2013 report
Do glial connectomes and activity maps make any sense?
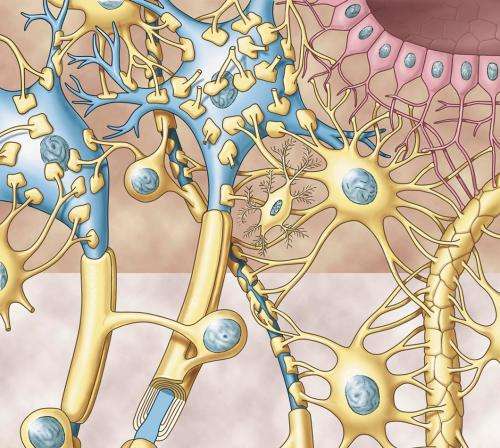
(Medical Xpress)—"If all you have is a hammer, everything looks like a nail." This so-called "law of the instrument" has shaped neuroscience to core. It can be rephrased as, if all you have a fancy voltmeter, everything looks like a transient electrical event. No one in the field understands this more Douglass Fields, an NIH researcher who has re-written every neuroscience dogma he has turned his scrupulous eye to. In a paper published yesterday in Nature, Fields questions the conventional wisdom that informs recent efforts to map the brain's connectivity, and ultimately, its electrical activity. In particular, he questions the value of making detailed maps of neurons, while at the same time neglecting the more abundant, and equally complex "maps" that exist for glia.
When first discovered, the "action potential" generated by a neuron was a rich and multiphysical event. It has since degenerated into a sterile, directionally-rectified electrical blip, whose only interesting parameter is a millisecond-scrutinized timestamp. In the last two years alone, Fields has re-generalized the spike. Having highlighted many of the fine scale physical events that accompany a neuron's firing, like temperature and volume changes, optical effects, displacement, and myriad nonsynaptic effects, Fields demonstrated the intimate knitting of reverse propagating spikes into the behavior and function of neuronal networks. He also showed how spikes directly control non-neuronal events, in particular, myelination.
The Eyewire project at MIT is a fantastic effort to create detailed neuronal maps—it expands neuroscience to the larger community, and generates much worthwhile scientific spin-off. It is also completely absurd. To have so much talk about brain maps without drawing clear distinction between the glaring contrast in the value of white matter maps and grey matter maps is telling. Maps of the white matter will be indespensible to understanding our own brains. They are highly personal, yet at the same time will be one of the most valuable things we might soon come to share. For the moment here, we can liken them to the subway or transportation map of a complex city.
To try and map the grey matter, at least in our foreseeable era, is to attempt to record the comings and goings of all the people entering and exiting the doors of the trains of our subway system. Not only is the task infinitely harder, pound for pound, it is equally less valuable, and impermanent. Looked at another way, if we imagine some hyper-detailed ecologist mapping the different trees in a forest, one valuable piece of information to have would be the tree species or type. Their age, size, density and distribution would similarly be worthwhile parameters. Also maybe some detail about their finer structure would be predictive of what kind of animals species might live and move about their arbors. Eyewire, on the other hand, is mapping every twig down to the finest termination as a leaf. The problem is that leaves are shed and regenerated anew each year, and while Eyewire might map a few neurons in the same time, synapses morph to a faster drum.
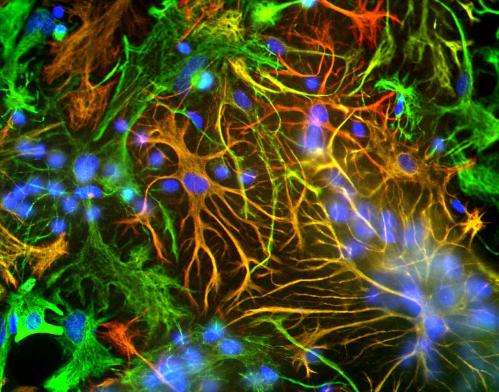
The point of Field's article is that glial trees have exactly the same level of detail and importance as neural trees, yet they are ignored in the aspirations of the connectomists. In fact, if neurons are like deciduous tress, with long, unpredictable, idiosyncratic and internexed branches, then glial cells, particularly astrocytes, are very much like conifers—they rigidly span nonoverlapping domains in the grey matter, in prototypical, scaffolded form, and with frequently symmetric repeatable structure. If we accept the results of neuroanatomy at face value here, grey matter might be imagined more like an astrocytic christmas tree farm superimposed on a neural rainforest. Stepping back, if given a choice between a grey matter connectome, and a white matter myelome, the latter is undoubtedly where the focus should be for now.
It may be a misstep in our study of glial cells to narrow-mindedly attempt to define for them, only that which has already been defined for neurons. The literature consists largely of a reattribution of transmitter or other chemical mechanisms of neurons to glia. The exceptioned qualifier here is that the speed of these processes—their electricality, directionality and extreme spatial aspect—is not a general feature of glial cells. For glial cells, new mechanisms need to be explored, and the most obvious among them perhaps, is that many of them, particularly the microglial cells, like to move.
It is increasingly appreciated nowadays, that much of the 10 or so watts attributed to the brain for its power budget, is purposed for things other then sending spikes and maintaining static electrical potentials. In the home, we can save on energy by dimming the lights, but to really make a dent, we need to turn off the things that move—things like fans, or the pumps in the HVAC systems. Much of the actual flow and motion inside the cerebral hive is transduced through glial cells. Undoubtedly axons drag diluent down their extent as they transport organelles across improbably expanses, and expel pressurized boluses of irritant (there may in fact be much to be said for an analogy with leaves powering fluid conduction in trees through local evaporation). It is however, the glial cells that seem to be the heavy lifters involved in flow. Transducing hand-picked intracellular flow, and bulk extracellular flow, sourced from the vasculature to neurons, they complete the so-called glymphatic circuit.
To be strict, perhaps we need to refigure this estimate of 10 watts, expanding it to include non-chemical sources, like the input of hydraulic power into the brain via the heart. If, for example, the brain consumes 20% of the flow from the heart, it also dissipates around 20% of the 100 or more watts of power generated by the heart. That should in fact be a significant contribution. By some estimates, we may have around 100,000 miles of myelinated axons in our brains, all surrounded by glial cells. Similarly, we may have the same amount, 100,000 miles, of capillary in the brain, all surrounded by astrocytic endfeet. Considering the scale of these numbers, it may be useful to start to look at the brain as more of a fluid-transporting machine, as opposed to mainly an electrical device.
The evidence is fairly clear that at the sensory and motor levels, spikes conduct much of the information about a stimulus or movement, particularly the short time scale components of that information. In moving more centrally from both sensory and motor ends, spikes tend to unhinge from real world metrics. If we are not careful to consider what neurons might actually be doing at a more global, physiologic level when they generate and propagate spikes, we may find that while we believe we are recording signals, we are actually just recording the noise of the pumps.
More information: Neuroscience: Map the other brain, www.nature.com/news/neuroscien … -other-brain-1.13654
© 2013 Medical Xpress