November 29, 2013 feature
Visualize this: Automated UV laser microsurgery simplifies microscopy and neurophysiology experiments in live animals
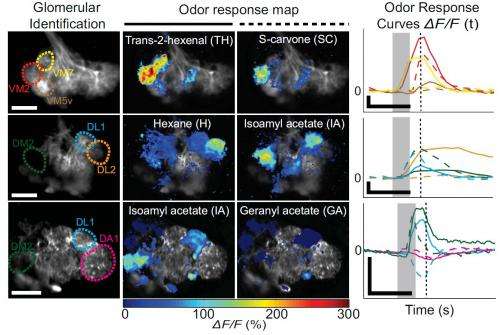
(Medical Xpress)—Although in vivo microscopy is a vital tool for monitoring cellular and neurophysiological processes, preparing live animals for microsurgery has traditionally had several significant limitations – namely, it takes time, requires a great deal of skill, and places constraints on what can be experimentally accomplished. Recently, however, scientists at Stanford University devised a largely automated protocol that addresses these limitations, and can be used in both optical and electrophysiological studies, by employing a highly precise pulsed excimer UV laser. The researchers not only used fruit flies as a model, but also demonstrate their technique in nematodes, ants, and the mouse cranium. Moreover, they see their findings as being of great value in neuroscience for investigating neuronal plasticity, learning, and memory.
Dr. Supriyo Sinha discussed the paper that he, Dr. Thomas M. Baer, Prof. Mark J. Schnitzer, and their co-authors published in Proceedings of the National Academy of Sciences. "A key challenge was identifying the optimal pulsed UV laser characteristics," Sinha tells Medical Xpress. "The laser pulse parameters must be chosen carefully, because the cuticle must be removed without damaging the fly's brain by applying excessive heat. Furthermore," he continues, "since we're monitoring neural activity by measuring the fluorescence from genetically encoded calcium indicators, it's important that the surgical laser pulses do not decrease the signal strength from these molecules as a result of, for example, photobleaching. Additionally, for high-throughput applications, the laser pulse parameters had to be selected such that the cuticle is removed quickly."
There were several steps in identifying the optimal pulsed laser. "We first identified the optimal laser wavelength. To minimize photobleaching, we selected a wavelength with a very short absorption length so that the light would not penetrate into the underlying brain." Since the fly cuticle is largely composed of water, the team chose a 193 nm wavelength because its absorption length in water is less than 1 micron. "We also tested more established UV laser systems that operate at the longer wavelength of 355 nm and confirmed the appearance of photobleaching – but we didn't measure any photobleaching at 193 nm."
When considering pulse parameters, the researchers used high-energy photons, nanosecond pulses and low-repetition rates to minimize heating. "We tested the imaging quality and the fly's behavior at different repetition rates and number of pulses to determine the optimal set of parameters. Finally, the fact that 193-nm pulsed lasers are available with high pulse energies allows us to remove the cuticle without scanning the laser beam, which decreases the surgical time considerably – by up to a few orders of magnitude depending on the area of cuticle to be removed – compared to traditional infrared ultrafast laser systems."
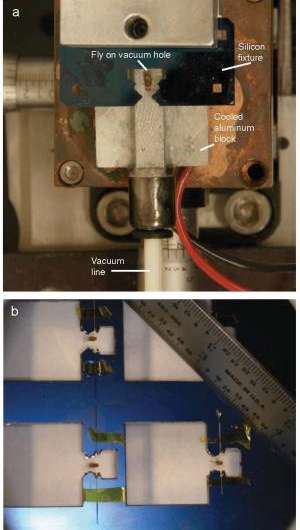
The scientists are also grappling with being able to remove the fly's perineural sheath without damaging underlying brain structure – a factor essential to conducting electrophysiological studies. As described above, the axial resolution of the measurement system – which would likely be interferometric – would have to be very high. "In addition, Sinha notes, "if a large section of sheath needs to be removed such that the amount of tissue between the sheath and the fly cuticle surface varies across the aperture, it's likely that we'd need to adjust the intensity appropriately across the profile. For a small section of sheath, this would not be an issue."
While they've not yet demonstrated successful removal of the perineural sheath, Sinha continues, the scientists have come up with a novel design that will allow them to generate a grey scale mask with an engineerable profile for our laser pulses – and grey scale masks are not trivial at this short UV wavelength. "We believe that this mask, coupled with spot scanning and real-time monitoring of cut through interferometric methods such as optical coherence tomography would allow us to reliably and repeatably remove the perineural sheath without damaging the underlying brain." Optical coherence tomography (OCT) is an optical signal acquisition and processing method that captures micrometer-resolution, three-dimensional images from within optical scattering media such as biological tissue.
Another challenge will be integrating cutting depth interferometric measurements into the surgical process. (Interferometric techniques measure small displacements, refractive index changes and surface irregularities.) "If the user simply wants to use interferometry to measure the profile of the cut after surgery, the integration would be fairly straightforward," Sinha points out. "However, the more interesting option would be to have a closed feedback loop that the system software would use to modify the surgical recipe in real time." The challenge of this approach, he adds, is that the interferometry data acquisition, data analysis and control electronics would have to have an extremely rapid refresh rate of roughly 100 Hz if very high resolution is desired, because the laser surgery component is only about 1 second in duration.
Regarding neuroscience applications beyond neuronal plasticity, learning and memory, Sinha says that a primary application of their laser microsurgery approach is to use it in a high-throughput system that could be used to reverse engineer neural circuits. "Efficient reverse engineering of circuits, whether electrical or biological, requires two things: A way to deterministically alter a single element in the circuit, and a method for quickly measuring system output for a given set of inputs," Sinha says. "The first is satisfied by the sophisticated genetic toolkit of fruit flies that allows targeting of single neurons. "We're working to develop the high throughput system to satisfy the second – and our laser microsurgery approach is an important component of that system."
Sinha points out that there is nothing inherently brain-specific about our microsurgery. "The precise nature of the surgery allows this approach to be a tool to study areas such as wound healing, in which various drugs could be screened to determine which of them work best to treat different kinds of wounds." While Sinha notes that their techniques may not be directly applicable to human translational and clinical neurological and neurosurgical applications, the researchers plan to use their approach different animal model organisms.
An emerging trend that speaks to the need for speed, precision and automation is that of robotic methods for handling and mounting flies or other small organisms that, when combined with the researchers' microsurgical techniques, should enable automated high-throughput preparation of live animals for optical experimentation. "These robotic methods, which include machine vision algorithms that accurately select and position flies with a high degree of accuracy, would be the first step in a high-throughput system," Sinha says, adding that a paper on this topic authored by other members in Prof. Schnitzer's group is in preparation.
Going forward, Sinha tells Medical Xpress, the main additional innovations they hope to incorporate in the laser microsurgery system are real-time surgical window monitoring and the ability to shape the laser beam intensity profile to allow for more controlled cuticle removal in the axial direction. Moreover, he adds, moving to red-shifted and "brighter" calcium indicators would also more easily allow for imaging through thinned cuticles.
"We're moving to brighter calcium indicators such as GCaMP6 (a genetically encoded calcium indicator designed to show the calcium ion status of tissue or other medium) that will better tolerate the presence of a thin layer of cuticle when imaging with cellular resolution," Sinha explains. "We're also looking at moving our two-photon excitation wavelength further into the infrared, which would substantially reduce scattering." The scientists also believe that by characterizing the fly cuticle at different ages, and through rearing flies in very repeatable conditions - reduced crowding, fresh food, humidity, temperature, and so on – it might be possible to thin the cuticle sufficiently without requiring feedback from interferometric techniques.
"The laser surgical system is a critical next step in a larger system that aims to image the brains of dozens of behaving fruit flies simultaneously," Sinha continues, mentioning that they allude to this somewhat obliquely in their paper. "Our demonstration of the four-fly mount and rapid surgery on the four flies that we reported is another step towards this system. In addition to robotic fly handling, we're also working on fly mounts that can hold a dozen flies, and have created a very compact footprint two-photon microscope that would allow ~100 fully functional independent two-photon microscopes to fit on a single optical table. We're also developing novel, efficient and compact laser light sources to allow dozens of flies to be imaged with two-photon microscopy simultaneously. Finally," he concludes, "we're also developing data acquisition and control electronics to control this array of two-photon microscopes and collect the hundred data streams."
Other areas of research might also benefit from their study. "In addition to neurological and non-neurological fly applications, our system could be used for other animals. For example," Sinha illustrates, "as we demonstrate in the paper, our approach could be used for mouse electrophysiology with multi-electrode devices." The researchers also think that the laser could be used in mice for creating optical windows and, possibly, thinned skull preparations.
To further extend the duration of time-lapse experiments, the researchers are also working on creating a preparation in which the fly cuticle is left entirely unopened and merely thinned for imaging. The key here, Sinha points out, is to maintain cellular or subcellular resolution of brain structure. "It's important that the cuticle is sufficiently thinned such that cuticle scattering does not greatly degrade the optical properties of the imaging system."
More information: High-speed laser microsurgery of alert fruit flies for fluorescence imaging of neural activity, PNAS Published online before print October 28, 2013, doi:10.1073/pnas.1216287110
© 2013 Medical Xpress. All rights reserved.