New weapons against antibiotic-resistant pathogens
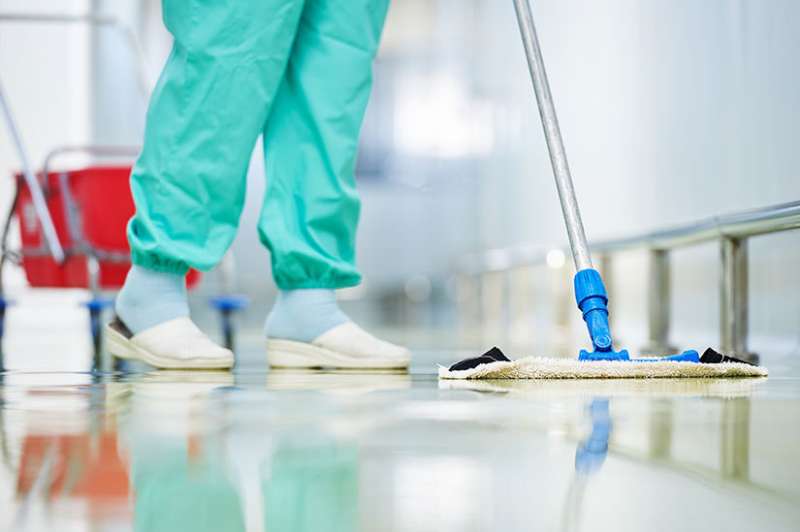
Alexander Fleming discovered penicillin in 1928—and arguably changed the course of history as the drug not only saved thousands of lives during World War II, but also laid the foundation for the antibiotic era that gave us decades of relative protection from previously deadly diseases.
Not even a century later, our excessive use of these miracle antibiotics has turned the tide against us and requires a renewed search for drugs that target the same kinds of bugs we've known for centuries. Now, however, they've become "superbugs," due to the genetic resistance they have developed against our original line of defense.
For the World Health Organization, antibiotic resistance is one of the biggest threats to global health and food security today, and researchers in the College of Engineering are helping to reduce that threat by limiting the spread of superbugs in hospitals and by developing new drugs that are less likely to elicit microbial resistance.
Containing hospital-acquired infections
Industrial and Systems Engineering Professor Oguzhan Alagoz first became interested in infectious diseases in 2009 when physician Nasia Safdar in the UW School of Medicine and Public Health alerted him to the bacterium Clostridium difficile, which often infects the colon of patients after they have been admitted to the hospital. It's a growing concern, both for her patients in Madison and for many others around the world.
These diarrhea-causing colon infections can be deadly for the already compromised immune system of patients undergoing cancer chemotherapy or receiving an organ transplant. The emergence of several drug-resistant C. diff. strains has contributed to an alarming increase in disease during the past decade, with almost half a million estimated infections and 30,000 deaths in 2011.
For Alagoz, the weapon of choice against C. diff. is an agent-based simulation: a mathematical model that mimics its transmission and identifies the best interventions for containing its spread—all while being much less expensive than a clinical trial. The "agents" in the model are hypothetical patients, doctors, nurses and visitors who interact with each other in rooms that may also become contaminated with C. diff.
Programmed by MD/Ph.D. student Anna Barker, whose advisors are Alagoz and Safdar, a network of computers simulates the movement of these agents within their environment during the course of a year and reflects the complex reality of an actual hospital much better than traditional epidemiologic models.
The simulations rely on data from previous studies and recent hospital admissions records, such as the length of a patient's stay; the frequency and duration of interactions between patients, healthcare workers and visitors; the probability of C. diff. transmission during person-to-person and person-to-environment contact; and the ability of nine different interventions, such as hand washing or room cleaning, to reduce transmission probabilities and limit the spread of C. diff.
Armed with a plausible range for these quantities, the model generates millions of possible infection paths and tests the effect of each intervention on these paths.
The results could be a game-changer: The model delivers estimated success rates (and their precision) for one intervention at a time and for any desired combination. "We found that the two most effective interventions were daily room cleaning with a sporicidal disinfectant and the C. diff. screening of patients' stool samples when they are first admitted to the hospital," Alagoz says.
Sporicidal disinfectants destroy the dormant and drug-resistant cells, or spores, that C. diff. forms to survive periods of nutrient deprivation, while the screening identifies patients who harbor the bacteria, but have not yet developed symptoms that would trigger existing intervention protocols.
Implemented together, these two strategies reduced hospital-onset infection by more than 80 percent in the simulations and were much more effective than visitor hand hygiene and contact precautions.
Alagoz and Safdar have submitted a grant proposal for follow-up work. The two most important next steps, they say, are to compare the model's predictions with actually observed infections and to identify the best sequence of interventions a hospital should implement, given its patient volume and budgetary constraints. "If our proposal is funded and our model can be validated, I believe it could be used in four to five years by any hospital in the country to contain the spread of C. diff. infections," Alagoz says.
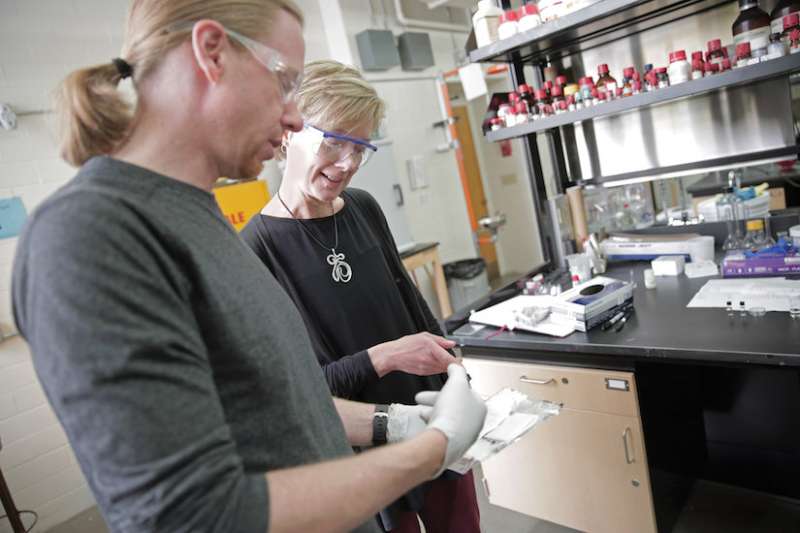
Designing next-generation antifungal drugs
Across the street from Alagoz's office, chemical engineers Sean Palecek and David Lynn have teamed up with Chemistry Professor Samuel Gellman to tackle the superbug problem from a basic science angle.
They are studying antimicrobial peptides, a promising class of pharmaceuticals that could make it more difficult for microbes to become drug-resistant. The researchers are currently focusing on fungi, whose cells are much more similar to our own than to those of bacterial and viral microbes.
"Because of this similarity, it's very challenging to develop selective antifungal drugs that aren't toxic to human cells," says Palecek, the Milton J. and A. Maude Shoemaker Professor in chemical and biological engineering.
Yet, the clinical demand for such drugs is high since hospital-acquired fungal infections have increased steadily since the early 1990s, especially in cancer and HIV/AIDS patients whose immune system is weakened by the drugs they receive and in organ transplant patients who are given immunosuppressants to prevent their bodies from rejecting their new organs.
Palecek's strategy is to engineer synthetic antimicrobial peptides. He aims to mimic the desirable properties of their naturally occurring counterparts while improving upon the undesirable ones, such as their chemical instability. His lab has used peptides extracted from the skin of Australian bell frogs as a starting point.
The frog's peptide and Palecek's synthetic version both target cell membranes. However, the synthetic peptide is designed to resist degradation by cellular enzymes and to disrupt the external membranes of fungal, but not human cells. Since the peptide interacts with multiple components on the fungal cell pores, the fungi are less likely to develop drug resistance.
"The better we can mimic the folded structure of native antimicrobial peptides, the more effective they seem to be, which is an encouraging finding," Palecek says.
While he tweaks the drug's chemical properties, David Lynn, the Duane H. and Dorothy M. Bluemke Professor and Vilas Distinguished Achievement Professor in chemical and biological engineering, focuses on delivering it only to the cells it is supposed to attack.
These cells typically gather on the surface of catheters and other medical devices. For example, Candida albicans, the most common fungal pathogen in humans, forms a biofilm on such devices that often gives rise to drug-resistant cells.
"Since the synthetic peptide is toxic, we can't just shoot it into a person's bloodstream," Palecek explains. "But if we coat a catheter with the special layer of chemicals that Dave's lab has developed before we put it in, a slow and sustained drug release at that specific site will kill the fungal calls while only a small amount will reach the rest of the body."
Alagoz relies on Safdar's clinical intuition to decide which variables to include in his agent-based model. Similarly, Palecek and Lynn collaborate with infectious disease physician David Andes in the UW School of Medicine and Public Health to evaluate their strategies in clinically relevant settings.
In 2016, Palecek, Lynn and Andes showed that coating a catheter with the slow-release antimicrobial peptide reduced the severity of C. albicans infection in a rat model. Andes says it is difficult to put an exact timeline on moving the research findings from the lab to the clinic, but believes that preclinical efficacy and safety studies will begin in the next few years.
The fight against microbes continues
Almost 100 years ago, Alexander Fleming's discovery of penicillin was serendipitous and it took 12 more years until Howard Florey and Ernst Chain proved its power to treat infectious diseases in 1940. The three scientists shared the Nobel Prize for these achievements in 1945.
Now that the superbugs have mounted their defense, the next stage of our battle against them requires a concerted effort on a global scale that unites engineers, physicians and many other researchers under the World Health Organization's global action plan on antimicrobial resistance.
The WHO's goal of continuing to treat and prevent infectious diseases with drugs that are used responsibly and available to all who need them is a tall order, but whether it's reached by systematic pursuit, serendipity or a combination of both, few stones will be left unturned along the way.