July 11, 2013 report
The brain is alive, will new MRI diffusion techniques let us see it move and shake?
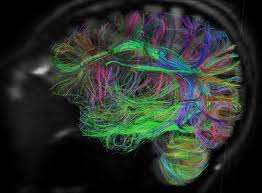
(Medical Xpress)—Pioneering experiments back in 1982 by Tasaki and Iwasa at the NIH revealed that action potentials in neurons are more than just the electrical blips that physiologists readily amplify and record. These so-called "spikes" are in fact multi-modal signalling packages that include mechanical and thermal disturbances propagating down the axon at their own rates. Nobel Laureate Francis Crick published a paper that same year, in which he postulated potential mechanisms that would explain twitching in dendritic spines, adding to an emerging picture of a brain more vibrant and motile than had been previously imagined. More recently, researchers have developed diffusion-based MRI methods, like diffusion tensor imaging (DTI), to trace the trajectories of axons, and perhaps more intriguingly, determine their directional polarity. Working at the EPFL in Switzerland, Denis Le Bihan and his co-workers have been using diffusional MRI in slightly different way. They now appear to be able to directly measure neuronal activity from the subtle movements of membranes, the water within them, and in the extracellular space around them. Their work, just published in PNAS, provides a much needed conceptual shift away from currently established, but typically nebulous, ideas regarding neurovascular coupling of brain activity to blood flow.
Present-day imaging methods, like blood oxygen level-dependent (BOLD) MRI, are only indirectly and remotely related to the cortical activity they often claim to measure. In 2006, Le Bihan reported a water "phase transition" response that preceded the neurovascular response normally detected by functional MRI. He attributed the changes in water diffusion to previously established effects involving membrane expansion and cell swelling secondary to activity. At the biophysical level, interpreting action potentials as phase transitions is a little off the beaten path from traditional neurobiology, but it can be an informative approach when to trying to understand what might be going on when cells fire.
As biophysicist Gerald Pollack has previously pointed out, spikes may involve the propagation of the line of transition of water from the ordered phase, (as patterned by hydrophic interactions nucleated at the surfaces of membranes and proteins) to a disordered phase.
Traditionally, the so-called bound surface water only extends out a only a couple of molecules from the surface of nondiffusable features. That idea may need to be revisited in light of more recent understanding when attempting to account for the diffusion of water in axons. A decrease in water diffusion as measured by MRI may be in part explained by a decrease in extracellular space, and that has been suggested from experiments measuring intrinsic optical effects. The larger picture of water diffusion, however, is likely a bit more complicated than this.
In his new study, Le Bihan stimulated the forepaw of a rat and looked at responses in the somatosensory cortex. The key experiment was to infuse nitroprusside in attempt to inhibit neurovascular coupling. It is a tricky alteration because nitroprusside apparently has many diffuse effects. It can induce potent vasodilation, particularly on the vascular end (mainly the smaller venules), after it breaks down to produce nitric oxide. It is also a diamagnetic molecule, and each molecule releases five cyanide ions, which are presumably detoxified by the mitochondrial enzyme rhodanese. The experiments were done under isoflurane anesthesia, which also introduces a few uncertainties, particularly with regard to responses to different frequencies of forepaw stimulation.
If nitroprusside is indeed a realistic experimental proxy for neurovascular uncoupling, then the results of Le Bihan appear to show that the diffusion response is not of vascular origin, and that it is closely linked to neural activation. He found that the standard BOLD MRI responses were completely quenched under nitroprusside, whereas the diffusion MRI responses were only slightly suppressed. Local field potentials were also simultaneously measured and suggested at least, that the neuronal responses were also intact.
The work of Le Bihan indicates that diffusion-based MRI can be used to infer neural activity directly from the structural changes that affect the molecular displacements of water. The ability to use shape changes in neurons, astrocytes, or even spines, raises the question of whether these kinds of techniques might eventually be of use in creating larger scale, and more detailed, Brain Activity Maps (BAMs). I asked Konrad Kording, author on the recent theoretical paper which discussed the theoretical limits to MRI and other activity recording methods, whether methods that probe water movements might be applied to this end.
Kording observed that the spatial resolution of standard MRI is ultimately limited by the diffusion of water, but more importantly perhaps, the temporal resolution of all known MRI methods is nowhere near that required to create spike maps. None-the-less, detecting mechanical responses in the brain could provide many unique insights into function. For example, experiments using agents that dissolve the extracellular matrix, like the clot-busting drug TPA, result in more twitching, or vibration if you will, in dendritic spines. Other studies have shown that the greater the electrical drive on a spine, the less it tends to twitch or change size, particularly during periods of rapid development.
Similarly, sensory deprivations appear to increase these kinds of movements as neurons grow and reorganize connections. While these effects are far below that which could be detected by any large external method of MRI, new tools may permit us to access these newly-revealed activities. Diffusional MRI in particular, can be done with a little modification of the standard MRI procedure. For example, to determine directional diffusion parameters, or diffusion tensors, typically six gradients are used to measure three directional vectors. As these capabilities become more common, hopefully the results of Le Bihan can be further explored and verified.
More information: Water diffusion in brain cortex closely tracks underlying neuronal activity, Published online before print June 25, 2013, doi: 10.1073/pnas.1303178110
Abstract
Neuronal activity results in a local increase in blood flow. This concept serves as the basis for functional MRI. Still, this approach remains indirect and may fail in situations interfering with the neurovascular coupling mechanisms (drugs, anesthesia). Here we establish that water molecular diffusion is directly modulated by underlying neuronal activity using a rat forepaw stimulation model under different conditions of neuronal stimulation and neurovascular coupling. Under nitroprusside infusion, a neurovascular-coupling inhibitor, the diffusion response and local field potentials were maintained, whereas the hemodynamic response was abolished. As diffusion MRI reflects interactions of water molecules with obstacles (e.g., cell membranes), the observed changes point to a dynamic modulation of the neural tissue structure upon activation, which remains to be investigated. These findings represent a significant shift in concept from the current electrochemical and neurovascular coupling principles used for brain imaging, and open unique avenues to investigate mechanisms underlying brain function.
© 2013 Phys.org