The brain's autofocus system helps stabilize vision despite motion
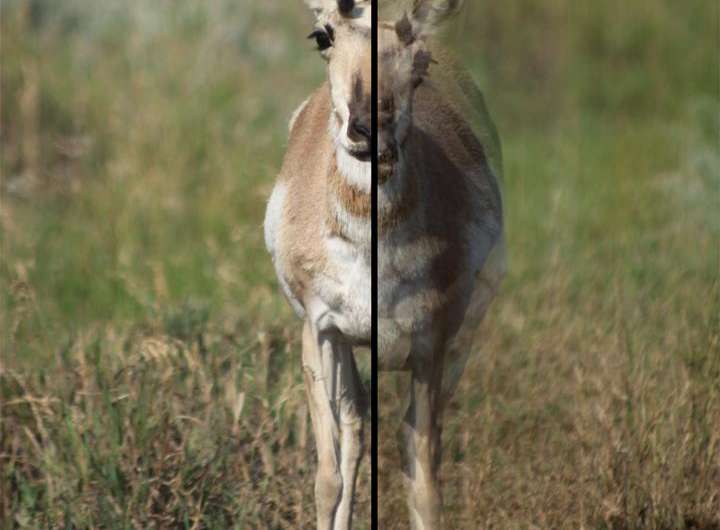
Much like the automatic focus of a camera, our eyes and brains must constantly recalibrate so that we can get a clear view of the changing—and always moving—world around us. Recently, two studies funded by the National Eye Institute (NEI) show how the circuitry for this eye-brain coordination is assembled during early embryonic development.
"Sensors in the eye detect movement and connect to the brain in just the right way to tell your eyes to move in the right direction without blurring images," said Andrew D. Huberman, Ph.D., of the University of California, San Diego School of Medicine, who led one of the new studies.
Say, for example, that you are running to catch your very fast, very small pet gerbil. The gerbil is moving and you are moving, so in order to focus on the gerbil, your eyes need a system to compensate for both sources of movement. This ability to compensate for movement is derived from the accessory optic system. The accessory optic system orchestrates the conversation between the brain and certain cells in the retina that detect movement, called direction-selective retinal ganglion cells (RGCs). Each direction-selective RGC is stimulated by visual motion in a specific direction and inhibited by motion in other directions.
Using mouse models, Huberman led a team demonstrating that two proteins, Contactin-4 and amyloid precursor protein, act during early development to establish and reinforce the accessory optic system.
Huberman and his team labeled specific target groups of neurons in the accessory optic system and then stained sections of the tissue with an antibody specific for Contactin-4. They found that those cells in the accessory optic system express (turn on) the Contactin-4 gene at the time that the system is developing.
Using a mouse model with a mutated Contactin-4 gene, they showed that Contactin-4's function is very specific to direction-selective RGCs. In those mice with mutated Contactin-4, the direction-selective RGCs in the eye didn't talk to the brain correctly. Likewise, in the absence of amyloid precursor protein, which binds to Contactin-4, normal eye and brain connections did not occur.
In another study coauthored by Huberman and led by Alex Kolodkin, Ph.D., of Johns Hopkins University, researchers describe further how direction-selective RGCs make precise, long-range connections to the brain.
From previous studies, Kolodkin and his group have shown that special proteins called semaphorins act as guides to help steer axons—the long tendrils of nerve cells—as they grow from the retina to their targets in the brain. But how these axons homed in on their target was still a mystery. In the current study, Kolodkin's group used mouse models to explore the function of another type of protein, called plexins. As it turns out, plexins act as kind of bait in the target region of the brain to attract RGC axons during early development. At the same time, semaphorins act as receptors on the RGC axons, allowing them to recognize the bait once they've reached it. Furthermore, Kolodkin's group found that the lack of correct eye-brain connectivity resulted in profound defects in image stabilization.
"Both investigations advance our understanding about how axons in the eye reach specific targets in the brain, which is an important step in developing new therapies for people with blinding eye diseases that affect the visual system, such as optic neuropathies or glaucoma," said Thomas Greenwell, Ph.D., Director of the NEI Retinal Neuroscience and Central Visual System Development and Regeneration programs.
Regenerating neurons in the eye and reconnecting them to the brain to restore vision is the cornerstone of NEI's Audacious Goals Initiative (AGI). As part of AGI, NEI held a workshop in Washington, D.C. on November 19, 2014 focused on optic nerve regeneration. Huberman was a participant and coauthor of a workshop summary, which describes strategies and challenges for developing new therapies to repair the optic nerve.
More information: Huberman AD et al. "Contactin-4 Mediates Axon-Target Specificity and Functional Development of the Accessory Optic System." Neuron, May 7, 2015. DOI: 10.1016/j.neuron.2015.04.005
Kolodkin AL et al. "Functional assembly of accessory optic system circuitry critical for compensatory eye movements." Neuron, May 7, 2015. DOI: 10.1016/j.neuron.2015.03.064