October 9, 2013 report
What happens when synapses run out of transmitter?
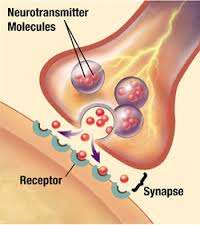
(Medical Xpress)—The recent Nobel Prize Award in Medicine highlights the importance of vesicle-based transport for different kinds of cells. One of the recipients, Thomas Sudhof, has contributed extensively to our current understanding of vesicle function in the synapses of neurons. Despite the fact that this is one of the most studied areas in neuroscience, we don't have a satisfactory theory that explains why information, ostensibly represented in high-fidelity using precisely timed spike trains, is then transferred with a low-fidelity, probabilistic mechanism that uses soft sacks of chemicals. A paper recently published in the journal Neuron, takes a closer look at this process in inhibitory neurons of the hippocampus. The authors find that if neurons continue to spike beyond a certain rate for a long enough time, their vesicles may still retain their potential for to fuse at release sites, but the synapse eventually runs out of transmitter to fill them with. Furthermore, they show that if the synapse can not supply sufficient transmitter, either through transport from the soma, or through local metabolic processing and uptake, then the synapse adapts by reducing the number of cycling vesicles.
We might imagine spikes, or other impulses in neurites, to be like electrical sparks. We can also imagine the vesicles as buckets. Excitatory synapses would then have their buckets filled with a kind of fuel that adds to the fire, while inhibitory buckets are filled with water that can douse it. The researchers here were looking at how buckets are filled with the inhibitory neurotransmitter GABA. They were able to control the availability of GABA at the synapse by manipulating the concentration of its amino acid precursors in the bath in which the cells were recorded. They could also block specific transporters which pump GABA into the buckets, and they could re-supply GABA directly to the cell through their recording pipet.
The basic protocol was to stimulate the presynaptic cell at a rate of 4 hz for as long as it took to see signs of "rundown" in simultaneously recorded postsynaptic cells. Usually, after about 500 to 2000 stimulus pulses, currents recorded in the postsynaptic cell would be considerably reduced. For a given synapse in the hippocampus, one typically sees that the probability that a spike causes a vesicle fusion, and subsequent postsynaptic spark, is only around one-third. Release probability is difficult to measure directly for a single synapse. Researchers sometimes apply models (typically based on binomial distributions) that were originally developed for the synapses between nerves and muscles, to synapses in the central nervous system. They often have limited applicability, particular among synapses that operate much differently from those at the neuromuscular junction. Some synapses, like the large ribbon synapse in the retina, might be able to support relatively high spontaneous spike rates throughout much of the day. In this case, several vesicles might be expected to fuse with each incoming spike, and fusions will correspondingly tend to occur even when there are no spikes as a direct casualty of high release probability.
I asked corresponding French author Stephane Supplisson what the release probability might be for the neurons he was studying. He indicated that when recording naturally occurring spikes in the presynaptic neuron, short-latency postsynaptic activity was pretty much guaranteed to be seen. This indicates that in these cultured neurons there are likely many synaptic contacts, and corresponding release sites, between any two connected cells. We might then ask, exactly what kind of hardware is it that the brain is using here, and for how long might it be reasonably overclocked?
One conclusion is cells prefer to use unreliable, but maximally flexible, synapses to exert on themselves upon their neighbors, provided they can pepper them with enough synapses to ensure the fidelity of their message. A synced message distributed across the dendritic tree of the postsynaptic cell would then pay for its initial wiring and maintenance costs by the added benefit of cooperatively drawing into play different sets of neurons which help amplify any signals local to a particular synapse. Each synapse thereby gives its all so to speak, at every spike or thought, however depending on their local environment and mitochondrial investment, synapses will likely exhaust themselves at different rates.
We might just note here that a hardware architecture where a neuron hits up each of its synapses on every spike bears analogy at a level of abstraction one above neurons. A brain whose neuronal processing units offer an opinion or response to everything that it can, would be the polar opposite to one built from Grandmother cells, or neurons with otherwise rigidly defined receptive or concept fields.
The authors do not yet have the full explanation as to how neurons, and their synapses, are be able to mothball empty vesicles in GABA-depleted synapses. Considering that a substantial portion of the energetic cost of neurotransmission is incurred at the synapse, when you reach a point where you can't fill your buckets there with water, then it would seem to make little practical sense to continue throwing them onto the flames.
More information: Cytosolic Transmitter Concentration Regulates Vesicle Cycling at Hippocampal GABAergic Terminals, Neuron, Volume 80, Issue 1, 143-158, 2 October 2013. DOI: 10.1016/j.neuron.2013.07.021
Abstract
Sustained synaptic transmission requires vesicle recycling and refilling with transmitter, two processes considered to proceed independently. Contrary to this assumption, we show here that depletion of cytosolic transmitter at GABAergic synapses reversibly reduces the number of recycling vesicles. Using paired recordings in hippocampal cultures, we show that repetitive activity causes two phases of reduction of the postsynaptic response. The first involves the classical depletion of the readily releasable and recycling pools, while the second reflects impairment of vesicle filling as GABA is consumed, since it can only be reversed by uptake of GABA or its precursors, glutamate or glutamine. Surprisingly, this second phase is associated with reduced quantal release, a faster depression rate and lower FM5-95 labeling, suggesting that the size of the cycling vesicular pool is regulated by cytosolic transmitter availability. Regulation of vesicular cycling may represent a general mechanism of presynaptic plasticity, matching synaptic release to transmitter supply.
© 2013 Medical Xpress