April 9, 2014 report
Controlling individual neurons with your brain
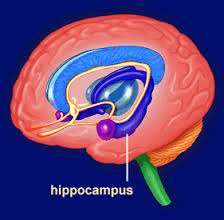
(Medical Xpress)—In many ways humans are universal machines. We can generate an answer to any question asked of us or move our body into any configuration our joints permit. We can do this near-instantaneously, and with semi-unconscious ease. While it is accepted that our neurons control these functions, we don't know if we can control the activity of individual neurons with this same ease and reliability. If we do possess this ability, then can we control the activity of any and every neuron, or just that of a few kingpins at critical nodes in our networks?
The activity or firing rate of a neuron depends on two things in particular—the synaptic input to the cell, and the cell's own programming of itself. Incredibly, a group of researchers from Japan have provided the first evidence that an animal (a mouse), can rapidly learn to reinforce patterns of synaptic activity in single neurons. In other words, once the researchers choose a particular cell, the mouse could route activity to it in such a way that it could receive a reward. The reward in this case was a highly-coveted electrical stimulation pulse to the lateral hypothalamus. This sweet spot in the brain's heart integrates information from the entire organism to control feeding, in particular, the desire for it. Stimulating it artificially seems to generate the "everything is awesome" note for the mouse.
Almost 50 years ago, researchers demonstrated a similar neurofeedback situation is possible to create in the motor cortex of a monkey. By using operant conditioning, the spike rates of single neurons could be more-or-less trained. Similar results have also been obtained for neurons in the medial temporal lobe of humans. What sets the the new studies apart, is that the feedback occurs entirely within the animals brain itself and therefore is not correlated to any degree with real world features or reward. Rather than requiring hours or days of training to see an effect, hypothalamic stimulation yields results within 15 minutes. Furthermore, the previous studies could not observe the finer scale synaptic dynamics that in large part control firing.
The Japanese researchers were able to measure the cumulative effects of the smaller "EPSPs" that occur in the dendrites of the cell. They did this in lightly or non-anesthetized, but head-fixed mice by patch-clamping directly onto individual neurons in the CA1 area of their hippocampus. When the measured activity met certain threshold criteria, the mice were conditioned with the hypothalamic reward. The researchers first identified CA1 neurons according to pre-established electrophysiological criteria. Namely, the cells had to fire bursts of three or more spikes at a rate of over 100 hz at least a few times a minute.
Although the mechanisms underlying this kind of burst spiking have been well-studied, they are still incompletely understood. In many cells they occur only after sufficient periods of quiessence, and then only when accompanied by selective calcium ion currents. While the researchers used this phenomena primarily to identify cell targets, they were also able to condition and de-condition these burst patterns according to the reward presence. That this capability of an animal to rapidly potentiate cell responses has broad implications for building brain-computer-interfaces should not escape us. To this point, studies just published for humans have shown that activity in a region of the brain called the nucleus accumbens (NAcc) can be voluntarily increased using neurofeedback.
Unfortunately the human NAcc activity was only controllable in the positive direction, ie. increased activity, and was just seen as a more transient (several seconds) fMRI effect. To actually start to use this kind of ability to control things in the external world, or even in the internal world of thought and memory, a greater understanding of how individual CA 1 cells fit into the larger ongoing activity of the hippocampus is needed. The researchers took several steps in this direction by looking at the relation of conditioning effects to local field potentials that represent larger groups of simultaneously active neurons. They noted that interneurons, while generally believed to be less plastic than the pyramidal-type neurons, seem to play a key role in shaping the background ripple potentials.
Additionally, the effects of different inputs to the cells, like the contributions of NMDA and dopamine receptors were studied. It was found that the ability to control neuron activity was decreased by stress, and by the researcher's conception of a depressed mouse state. The neuron-warping, reality distortion field effects, were however apparently restored by the antidepressant drug fluoxetine. For me personally, the use of stressors—like the treading-water survival test or immobilization—add nothing the interesting results hither-to obtained. The same for the obligatory disease link and rescue effects. Despite their continued appeal to many researchers, these methodical, perfunctory, protocols have gotten tired. I think we need hope that neuroscience can eventually move beyond them in uncovering new phenomena.
More information: Operant Conditioning of Synaptic and Spiking Activity Patterns in Single Hippocampal Neurons, The Journal of Neuroscience, 2 April 2014, 34(14): 5044-5053; DOI: 10.1523/JNEUROSCI.5298-13.2014 . www.jneurosci.org/content/34/14/5044
Abstract
Learning is a process of plastic adaptation through which a neural circuit generates a more preferable outcome; however, at a microscopic level, little is known about how synaptic activity is patterned into a desired configuration. Here, we report that animals can generate a specific form of synaptic activity in a given neuron in the hippocampus. In awake, head-restricted mice, we applied electrical stimulation to the lateral hypothalamus, a reward-associated brain region, when whole-cell patch-clamped CA1 neurons exhibited spontaneous synaptic activity that met preset criteria. Within 15 min, the mice learned to generate frequently the excitatory synaptic input pattern that satisfied the criteria. This reinforcement learning of synaptic activity was not observed for inhibitory input patterns. When a burst unit activity pattern was conditioned in paired and nonpaired paradigms, the frequency of burst-spiking events increased and decreased, respectively. The burst reinforcement occurred in the conditioned neuron but not in other adjacent neurons; however, ripple field oscillations were concomitantly reinforced. Neural conditioning depended on activation of NMDA receptors and dopamine D1 receptors. Acutely stressed mice and depression model mice that were subjected to forced swimming failed to exhibit the neural conditioning. This learning deficit was rescued by repetitive treatment with fluoxetine, an antidepressant. Therefore, internally motivated animals are capable of routing an ongoing action potential series into a specific neural pathway of the hippocampal network.
© 2014 Medical Xpress